Breastfeeding boosts metabolites important for brain growth
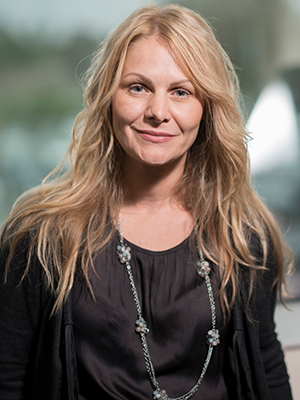
“Proton magnetic resonance spectroscopy, a non-invasive imaging technique that describes the chemical composition of specific brain structures, enables us to measure metabolites that may play a critical role for growth and explain what makes breastfeeding beneficial for newborns’ developing brains,” says Catherine Limperopoulos, Ph.D.
Micro-preemies who primarily consume breast milk have significantly higher levels of metabolites important for brain growth and development, according to sophisticated imaging conducted by an interdisciplinary research team at Children’s National.
“Our previous research established that vulnerable preterm infants who are fed breast milk early in life have improved brain growth and neurodevelopmental outcomes. It was unclear what makes breastfeeding so beneficial for newborns’ developing brains,” says Catherine Limperopoulos, Ph.D., director of MRI Research of the Developing Brain at Children’s National. “Proton magnetic resonance spectroscopy, a non-invasive imaging technique that describes the chemical composition of specific brain structures, enables us to measure metabolites essential for growth and answer that lingering question.”
According to the Centers for Disease Control and Prevention, about 1 in 10 U.S. infants is born preterm. The Children’s research team presented their findings during the Pediatric Academic Societies 2019 Annual Meeting.
The research-clinicians enrolled babies who were very low birthweight (less than 1,500 grams) and 32 weeks gestational age or younger at birth when they were admitted to Children’s neonatal intensive care unit in the first week of life. The team gathered data from the right frontal white matter and the cerebellum – a brain region that enables people to maintain balance and proper muscle coordination and that supports high-order cognitive functions.
Each chemical has its own a unique spectral fingerprint. The team generated light signatures for key metabolites and calculated the quantity of each metabolite. Of note:
- Cerebral white matter spectra showed significantly greater levels of inositol (a molecule similar to glucose) for babies fed breast milk, compared with babies fed formula.
- Cerebellar spectra had significantly greater creatine levels for breastfed babies compared with infants fed formula.
- And the percentage of days infants were fed breast milk was associated with significantly greater levels of both creatine and choline, a water soluble nutrient.
“Key metabolite levels ramp up during the times babies’ brains experience exponential growth,” says Katherine M. Ottolini, the study’s lead author. “Creatine facilitates recycling of ATP, the cell’s energy currency. Seeing greater quantities of this metabolite denotes more rapid changes and higher cellular maturation. Choline is a marker of cell membrane turnover; when new cells are generated, we see choline levels rise.”
Already, Children’s National leverages an array of imaging options that describe normal brain growth, which makes it easier to spot when fetal or neonatal brain development goes awry, enabling earlier intervention and more effective treatment. “Proton magnetic resonance spectroscopy may serve as an important additional tool to advance our understanding of how breastfeeding boosts neurodevelopment for preterm infants,” Limperopoulos adds.
Pediatric Academic Societies 2019 Annual Meeting presentation
- “Improved cerebral and cerebellar metabolism in breast milk-fed VLBW infants.”
- Monday, April 29, 2019, 3:30–3:45 p.m. (EST)
Katherine M. Ottolini, lead author; Nickie Andescavage, M.D., Attending, Neonatal-Perinatal Medicine and co-author; Kushal Kapse, research and development staff engineer and co-author; Sudeepta Basu, M.D., neonatologist and co-author; and Catherine Limperopoulos, Ph.D., director of MRI Research of the Developing Brain and senior author, all of Children’s National.