Study suggests chronic hypoxia delays cardiac maturation in CHD
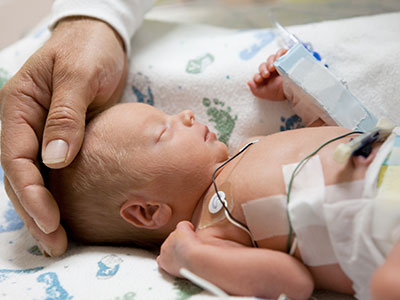
Every year, nearly 40,000 babies are born with a congenital heart defect (CHD) — the leading cause of birth defect-associated infant illness and death.
Every year, nearly 40,000 babies are born with a congenital heart defect (CHD) — the leading cause of birth defect-associated infant illness and death. An event that may contribute to cyanotic CHD is the lack of oxygen, known as hypoxia, before and after birth, impacting gene expression and cardiac function that delay postnatal cardiac maturation, according to a new pre-clinical model led by researchers at Children’s National Hospital.
Single ventricle, transposition of the great arteries, truncus arteriosus and severe forms of tetralogy of Fallot, such cyanotic congenital heart diseases have lower circulating blood oxygen levels. The lack of oxygen in the blood begins prenatally and continues after birth until definitive repair, suggesting a delay on cardiac maturation.
There is little research on the underpinnings that explain the lack of oxygen’s effects on the developing heart, which could help inform adequate therapies in the pediatric population to promote cardiovascular health across the lifetime. The researchers developed the first pre-clinical model that explores the effects of chronic hypoxia in perinatal and postnatal stages on the developing heart under conditions seen in cyanotic CHD.
“To the best of our knowledge, ours is the first study to perform complete gene expression arrays on animals after perinatal hypoxia,” said Jennifer Romanowicz, senior noninvasive imaging fellow at Boston Children’s Hospital and lead author of the study. “Not only did these studies allow us to determine the effects of hypoxia on heart development, but the detailed results of our study will be available to other researchers to independently address other questions about perinatal hypoxia and heart development.”
The study published in the American Journal of Physiology: Heart and Circulatory Physiology suggests that chronic lack of oxygen alters the electrical properties of heart tissue, called the electrophysiological substrate, and the contractile apparatus, a muscle composed of proteins that control cardiac contraction. Multiple genes involved with the contractile apparatus were expressed differently in the non-human subjects.
“What was remarkable was that most abnormalities normalized after the animals recovered in normal oxygen levels,” said Romanowicz. “This is an optimistic sign that early repair of cyanotic congenital heart disease may allow the heart to finish development.”
The researchers placed pregnant non-human subjects in hypoxic chambers starting on embryonic day 16, mimicking the second trimester in humans. The same subjects gave birth in the hypoxic chambers, and the newborns were kept there until postnatal day eight when the heart muscle maturation is nearly complete. To understand how human infants recover with normalized oxygen levels after surgical repair of cyanotic CHD, the researchers moved hypoxic subjects to normal oxygen conditions for recovery and tested again at postnatal day 30.
“Next steps include using a pre-clinical model of cyanotic congenital heart disease that more accurately represents human neonatal physiology,” said Devon Guerrelli, Ph.D. candidate at Children’s National. We plan to work with the cardiac surgery team at Children’s National to investigate changes in the myocardium due to hypoxia in pediatric patients who are undergoing surgical repair.”
Nikki Posnack, Ph.D., principal investigator at Sheikh Zayed Institute for Pediatric Surgical Innovation and Nobuyuki Ishibashi, M.D., director of Cardiac Surgery Research Laboratory at Children’s National, led and guided the team of researchers involved in the study.