Study finds delayed oligodendrocyte progenitor maturation in Down syndrome
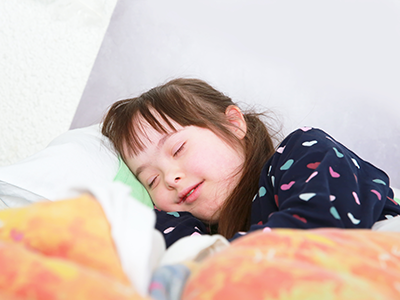
People with Down syndrome (DS) can have moderate to severe intellectual disability, which is thought to be associated with changes in early brain development.
People with Down syndrome (DS) can have moderate to severe intellectual disability, which is thought to be associated with changes in early brain development. Children’s National Hospital experts discovered delayed maturation in oligodendrocyte progenitors in DS. Oligodendrocytes produce the white matter which insulates neural pathways and ensures speedy electrical communication in the brain. The researchers identified these delays by measuring gene expression at key steps in cell development, according to a new study published in Frontiers in Cellular Neuroscience.
The findings further suggest that brain and spinal cord oligodendrocytes differ in their developmental trajectories and that “brain-like” oligodendrocyte progenitors were most different from control cells, indicating that oligodendrocytes in the brains of people with DS are not equally affected by the trisomy 21.
“This is one of the critical steps towards identifying the key stages and molecular players in the DS white matter deficits,” said Tarik Haydar, Ph.D., director of the Center for Neuroscience Research. “With this knowledge, and with further work in this direction, we envision future therapies that may improve nerve cell communication in the brains of people with Down syndrome.”
The hold-up in the field
The mechanisms that lead to the reduction of white matter in the brains of people with DS are unknown. To better understand early neural precursors, they used isogenic pluripotent stem cell lines derived from two individuals with Down syndrome to study the brain development and spinal cord oligodendrocytes.
“I was excited that we discovered another example of how important it is not to generalize when studying DS brain development,” said Haydar. “This is one of several papers, from our group and others, that demonstrate how important it is to be very specific about the brain area and the developmental stage when investigating the causes of DS brain dysfunction.”
What’s next
Dysmaturation of oligodendrocyte cells are a relatively new discovery by the Haydar Lab, one of the preeminent labs in DS research. These results isolate specific steps that are affected in human cells with trisomy 21. They are using these results to develop a drug screening platform that may prevent altered generation of oligodendrocytes in the future.
You can read the full study “Sonic Hedgehog Pathway Modulation Normalizes Expression of Olig2 in Rostrally Patterned NPCs With Trisomy 21” in Frontiers in Cellular Neuroscience.