Could whole-exome sequencing become a standard part of state newborn screening?
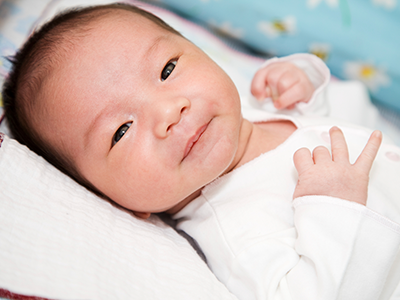
There are concerns about implementing whole-exome sequencing since it takes away the child’s right to decide if they want to know — or not — about their specific inherited disease.
It is still premature to standardize an innovative methodology known as whole-exome sequencing (WES) as part of state newborn screening programs, argues Beth A. Tarini, M.D., M.S., associate director for the Center of Translational Research at Children’s National Hospital, in a new editorial published in JAMA Pediatrics.
About 4 million infants are born annually in the United States. Newborn screening is a mandatory state-run public health program that screens infants for inherited diseases in the first days of life so they can receive treatment before irreversible damage occurs. Several of these screening tests are done on blood drawn from an infant’s heel.
WES holds the potential to screen infants for thousands of disorders and traits, including those that appear in adulthood. But there are concerns about implementing WES since it takes away the child’s right to decide if they want to know — or not — about their specific inherited disease. There is also the unknown effect that it could have on their ability to obtain health insurance.
“As caretakers for their children, parents have the challenge of deciding what kind of information, including genetic, will be valuable for their child,” says Dr. Tarini. “As a society, we have the responsibility of deciding where the healthcare dollars get the best return – especially when it comes to children. We need to start that conversation for universal genomic sequencing of newborns sooner rather than later.”
The Pereira et al. study, appearing in the new edition of JAMA Pediatrics and referenced in Dr. Tarini’s editorial, is the first to demonstrate no significant harm in the initial 10 months of life after performing WES under the best conditions of access to resources and a controlled environment.
While the Pereira et al. study has limited data on the effects of WES on families from underrepresented backgrounds, Dr. Tarini notes that it does provide a critical first step in this area of pediatric genomic research and for policy decision-making about the widespread implementation of WES in newborns.
“Moving forward, the U.S. will have to make a collective decision about the value of WES for newborns,” says Dr. Tarini. That value calculus cannot be made without consideration of the general state of healthcare for infants. As she points out, “This is not an easy question to answer in a country whose infant mortality ranks 34th according to the Organization for Economic Co-operation and Development (OECD).”
Dr. Tarini’s research identifies ways to optimize the delivery of genetic services to families and children, particularly newborn screening. She has also chaired state newborn screening committees and served on several federal newborn screening committees.