Dr. Nathan A. Smith receives $600,000 DOD ARO grant to study the role of glial cells in neural excitability and cognition
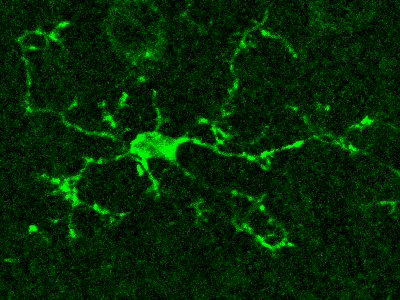
Microglia are the resident immune cells of the central nervous system that have highly dynamic processes that continuously survey the brain’s microenvironment, making contact with both neurons and astrocytes.
In his pursuit to understand the function of neural circuits within the brain, Nathan A. Smith, M.S., Ph.D., principal investigator at Children’s National Hospital, is examining how specialized glial cells, known as astrocytes and microglia, work together to influence neural networks and potentially enhance neuro-cognition.
Dr. Smith has just secured a new $600,000 grant from the Department of Defense Army Research Laboratory to pursue cutting-edge experimental approaches to examine the role of astrocytes in Ca2+-dependent microglia modulation of synaptic activity. This project will enhance our understanding of neuronal excitability and cognition, and define a new role for microglia in these processes.
“Glia cells play an important role in modulating synaptic function via Ca2+-dependent mechanisms,” says Dr. Smith. “It’s time for these cells to receive recognition as active participants, rather than passive contributors, in fundamental neural processes.”
Dr. Smith and his laboratory at Children’s National Research Institute are using novel experimental models to study the dynamics underlying Ca2+-mediated microglia process extension and retraction to further our understanding of how microglia, astrocytes and neurons interact in the healthy brain.
“Completion of the proposed studies has the potential to redefine the role(s) of microglia in higher brain functions and highlight the significant contribution of these cells,” Dr. Smith says. “Most importantly, elucidating the mechanisms that underlie glial cell modulation of neural circuits will not only further our understanding of normal brain function but also open new avenues to developing more accurate computational models of neural circuits.”
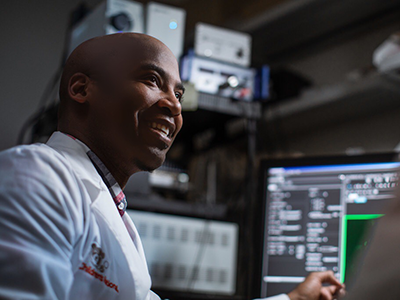
Dr. Smith and his laboratory at Children’s National Research Institute are using novel experimental models to study the dynamics underlying Ca2+-mediated microglia process extension and retraction to further our understanding of how microglia, astrocytes and neurons interact in the healthy brain.
Microglia are the resident immune cells of the central nervous system that have highly dynamic processes that continuously survey the brain’s microenvironment, making contact with both neurons and astrocytes. However, because of our inability to directly monitor Ca2+ activity in microglia, very little is known about the intracellular Ca2+ dynamics in resting microglia and their role in surveillance and modulation of synaptic activity.
Dr. Smith’s research team and his use of cutting-edge technology are a perfect match with the Army’s new modernization priorities. Dr. Smith’s research program and the new Army’s initiatives will greatly benefit from each other and ultimately contribute to a better understanding of the human brain.
“This research will help address a major gap in our understanding of the roles that glial cells play in regulating the computations of the nervous system through their interactions with neurons, which could also inspire a new class of artificial neural network architectures,” said Dr. Frederick Gregory, program manager, Army Research Office, an element of the U.S. Army Combat Capabilities Development Command’s Army Research Laboratory.
The grant will begin on July 1, 2020, and will last over three years. Dr. Smith’s research is also supported by other grants, including awards from the NIH and the National Science Foundation.
“As Dr. Smith’s mentor, the ultimate joy for a mentor is to see his mentees follow their dreams and be recognized for their accomplishments,” said Vittorio Gallo, Ph.D., Chief Research Officer at Children’s National Hospital. “I couldn’t be prouder of Nathan, and I am fully confident that this new research grant will help him continue to grow an exceptional research program.”